Introduction:
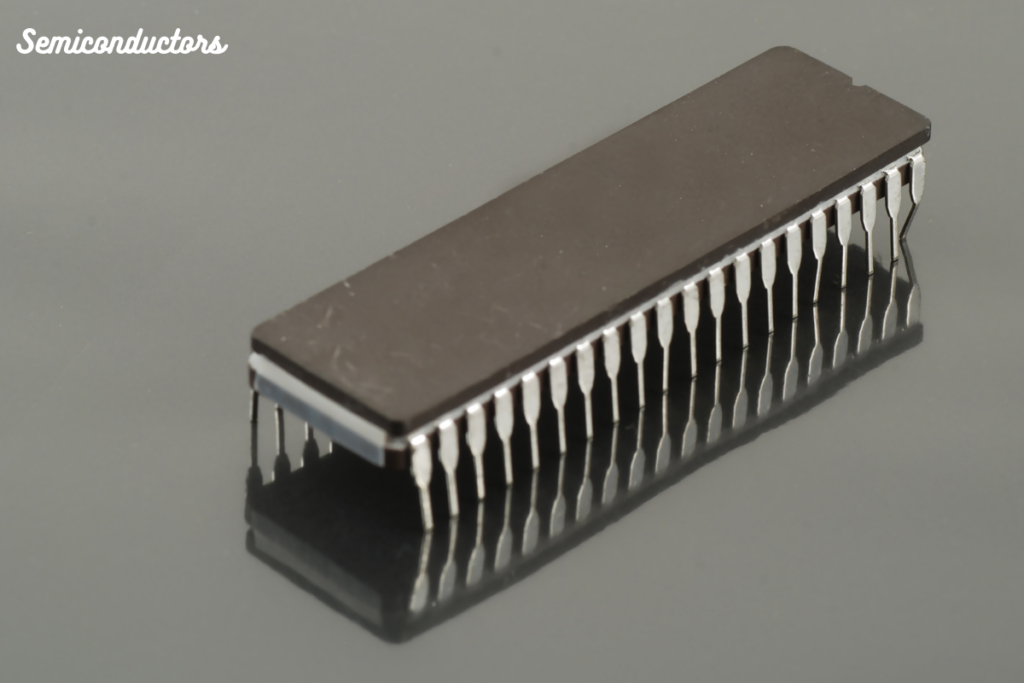
Many of the technologies of quantum computing are derived from “semiconductors,” which are also the basic materials driving their development. These materials have exactly controllable electrical characteristics that enable the building blocks of quantum information—qubits—to be created.
Many of the technologies of quantum computing are derived from Semiconductors, which are also the basic materials driving their development. These materials have exactly controllable electrical characteristics that enable the building blocks of quantum information—qubits—to be created. The special properties of these material allow effective control and interaction of qubits as quantum computers seek to execute computations at speeds much above those of conventional computers. “The Role of Semiconductors in Quantum Computing“ From materials research to cryptography, this capacity is important for creating scalable and robust quantum systems able to solve challenging challenges in many spheres.
The developments in quantum computing mostly depend on the discoveries about “Semiconductors”. Different semiconductor materials and architectures are under investigation by researchers to improve qubit dependability and performance. For example, new eras of computer capability are being ushered in by developments including topological qubits and superconducting “Material”. The need for “special material” in this transforming technology becomes increasingly more important as the demand for faster and more efficient quantum systems increases, therefore underlining their relevance in the continuous search for pragmatic and widely used quantum computing solutions.
Table of Contents
Foundation of Qubits:
The way qubits are generated and used has been transformed by including “semiconductors” into quantum computers. Using the special qualities of these materials allows scientists to effectively control quantum bits, hence enabling strong quantum technologies.
Qubit Creation:
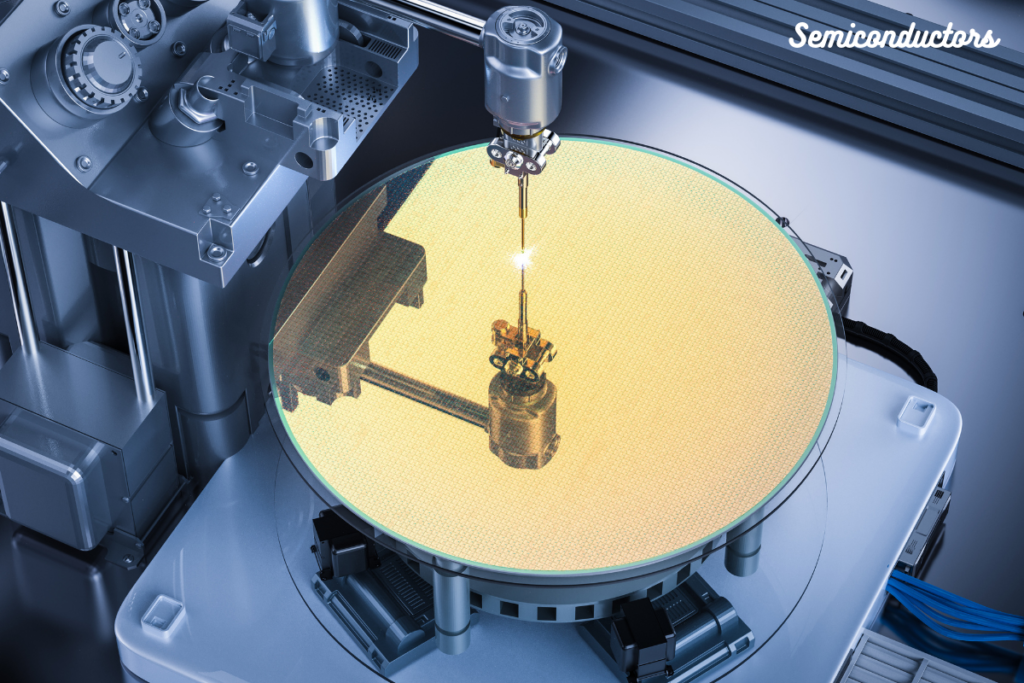
The idea of qubits—the basic units of quantum information—lies at the core of quantum computing. “Semiconductors” are quite important for one’s capacity to build, regulate, and manipulate these qubits. By use of precise electrical properties, these materials can be designed to show qubits capable of existence in superposition and entangled states. The complicated processes at the foundation of quantum computing are made possible by “these material’ inherent ability to host and control quantum states.”
Manipulation and Control:
Beyond only building qubits, “semiconductors” are absolutely essential for the manipulation and control of these quantum bits. Applying external electromagnetic fields helps researchers control the states of qubits generated from “this special material,” therefore enabling exact operations required for quantum computers. Quantum algorithms depend on this complex control since it allows actions including quantum gate implementations using the benefits of superposition and entanglement. Therefore, “this special material” not only form the basis of qubits but also help to enable the inventions required for the useful implementation of quantum computing.
Enhanced Control:
Stability and efficiency of qubits depend on the capacity to regulate the electrical states within quantum systems. Unique qualities of “semiconductors” allow exact control of these states, hence guaranteeing the integrity required for strong quantum computing uses.
Precision in Control:
The special qualities of “Semiconductors” make them indispensable in reaching great degrees of accuracy in management of electronic states. Crucially for controlling qubit states, these materials permit fine-tuning of energy levels and interactions between charge carriers. Researchers can efficiently control qubits by using “this unique material” by means of methods include quantum dot creation or superconducting circuits. Maintaining coherence depends on this precision since it reduces the impacts of noise and environmental disturbances that can cause decoherence, hence protecting the integrity of qubits throughout time.
Maintaining Qubit Integrity:
Within the field of quantum computing, the performance of intricate computations and algorithms depends critically on qubit integrity. “Semiconductors” are essential for maintaining stable and dependable qubit states in face of quantum system inherent difficulties. “Semiconductors” help reduce possible mistakes that can disturb quantum computing by allowing efficient error correction and real-time monitoring of electronic states. Therefore, the improved control given by “Semiconductors” not only increases the dependability of qubit operations but also increases the general practicality of quantum computing, thereby becoming “Semiconductors” a future-oriented prospective technology.
Material Diversity:
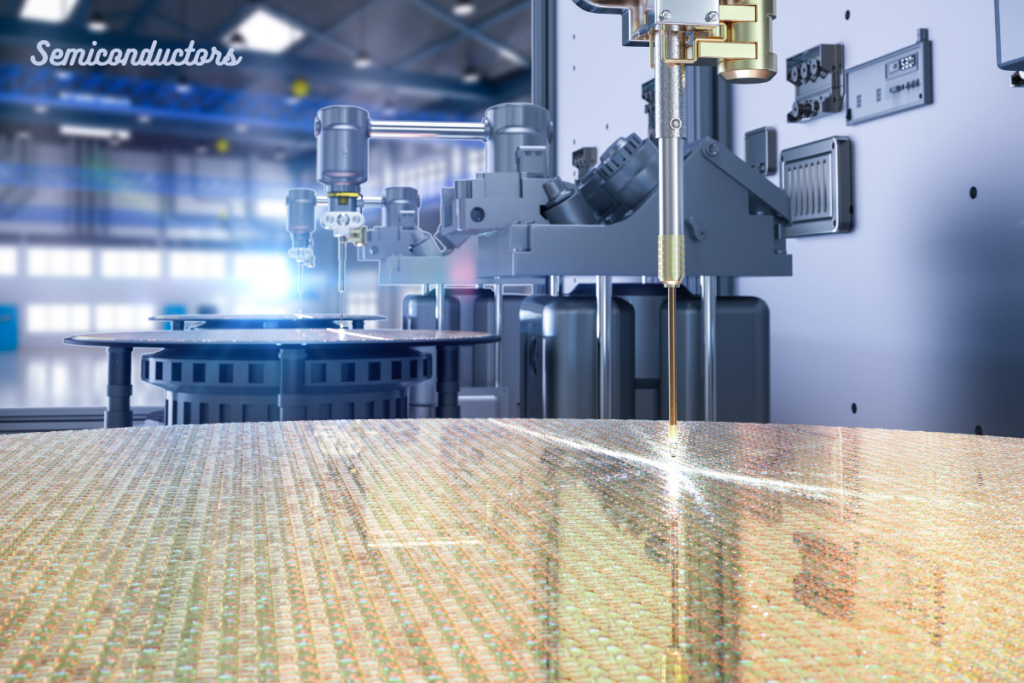
Qubit performance and scalability in quantum computing are substantially affected by the variety of materials applied in it. Aiming to maximize quantum systems for different uses, researchers are investigating several kinds of “Semiconductors” to exploit their special qualities
Types of Semiconductors:
The diversity of “Semiconductors” accessible—including conventional silicon and sophisticated superconducting materials—helps much in the field of quantum computing. Since silicon is the foundation of conventional electronics, it has been much investigated for fit with current technologies and manufacturing techniques. Conversely, as they can carry electrical current without resistance, superconducting “This Material” offer improved qubit performance. More strong quantum computers are made possible by researchers customizing qubit designs to maximize their efficiency and functionality by using the particular advantages given by various kinds of “coming out from these material”.
Optimizing Performance and Scalability:
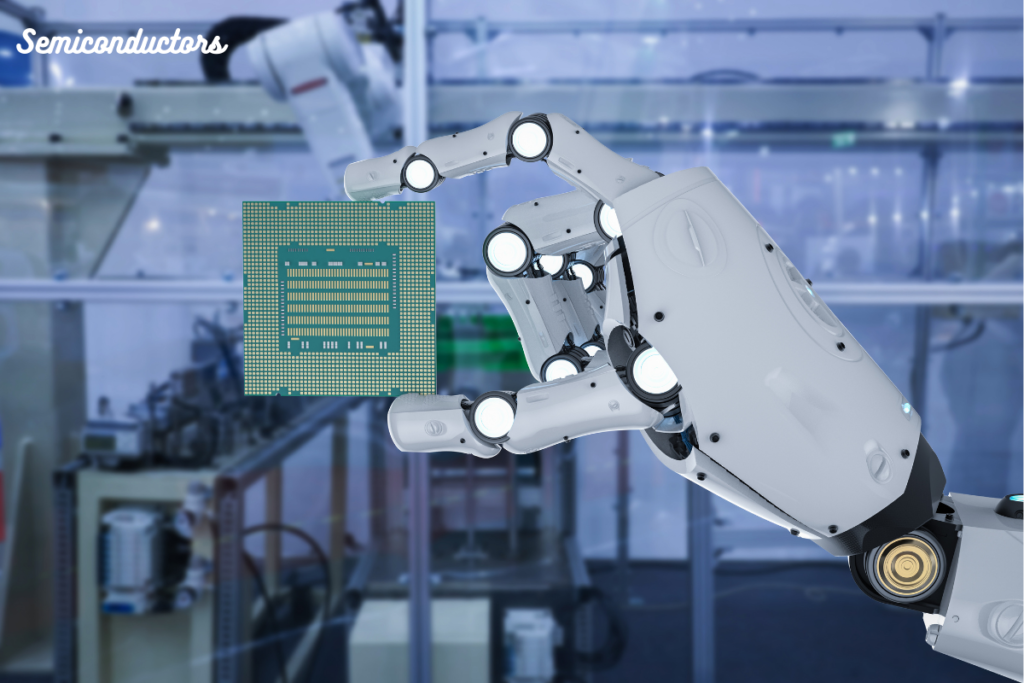
Dealing with the performance and scalability issues in quantum computing depends on the use of “Semiconductors”. Unique thermal, electrical, and physical characteristics of every kind of semiconductor material can affect operational speed and qubit coherence times. Constant investigation on novel materials, including topological “Semiconductors,” seeks to improve qubit scalability and durability. Combining the strengths of several “Semiconductors” aims to create scalable quantum systems that can effectively handle challenging computations while avoiding mistakes, hence stretching the limits of what quantum computing can accomplish.
Quantum Gate Operations:
Fundamental to the operation of quantum algorithms and allowing the completion of challenging computations are quantum gate operations. “Semiconductors” are crucial parts of quantum computing systems since their special properties help to enable these processes.
Building Blocks of Quantum Algorithms:
Like conventional logic gates, quantum gates run on qubits and control their states to execute computations. The application of these quantum gates mostly depends on the characteristics of “Semiconductors.” Different kinds of “Semiconductors” can be developed to produce particular kinds of gates, such Pauli gates and Hadamard gates, which are required for running several quantum algorithms.
Using “Semiconductors”, scientists can create circuits that use the quantum character of particles, therefore converting superposition and entanglement into efficient computing mechanisms. “The Future of Semiconductors: A Quantum Perspective” This integration of quantum principles with traditional semiconductor technology enhances the capabilities of quantum computing, paving the way for advancements in various applications.
Facilitating Complex Computations:
The function of “semiconductors” in quantum gate operations goes beyond simple application; they also help to effectively run difficult computations. “Semiconductors” can enable fast and dependable switching between qubit states, which is necessary for the quick execution of quantum processes because of their special electrical and thermal qualities. By lowering latency and error rates, thereby expediting the route toward actual quantum applications, “Semiconductors” not only helps to build effective quantum gates but also improves the general performance of quantum algorithms.
Error Correction:
Quantum computing depends critically on error correction since qubit states are sensitive to many kinds of faults due of their delicate character. Novel ideas in “Semiconductors” are opening the path for sophisticated quantum error correction methods, hence improving the dependability and stability of quantum computers.
Role of Semiconductors in Error Correction:
Using “semiconductors” in quantum computing helps to create strong error correcting systems meant to solve qubit instability’s problems. These materials can be designed to allow the synthesis of logical qubits—which combine several physical qubits to create a more dependable quantum state. Leveraging the special characteristics of “this special materail,” researchers can design error-correcting codes enabling the identification and rectification of decoherence- and operational noise-generated faults. Maintaining the integrity of quantum computing depends on this ability to guarantee exact results even in the presence of faults.
Enhancing Reliability and Performance:
Innovations in “Semiconductors” not only help to detect and rectify errors but also significantly increase the general quantum computer performance. Sophisticated control systems using “Semiconductors” allow researchers to track qubit states in real time and quickly apply required changes. This dynamic method of error correction greatly improves the dependability of quantum computation, hence enabling sophisticated algorithms needing a high degree of accuracy. The field of quantum error correction will change as “Semiconductors” advance, therefore fostering the creation of more sensible and scalable quantum computing systems able to solve problems in the real world.
Integration with Classical Systems:
Combining classical and quantum computing could open fresh possibilities and efficiency in many different fields. This hybrid development depends much on the compatibility of “Semiconductors” with conventional computing systems, therefore fostering a flawless integration of both technologies.
Compatibility with Classical Architectures:
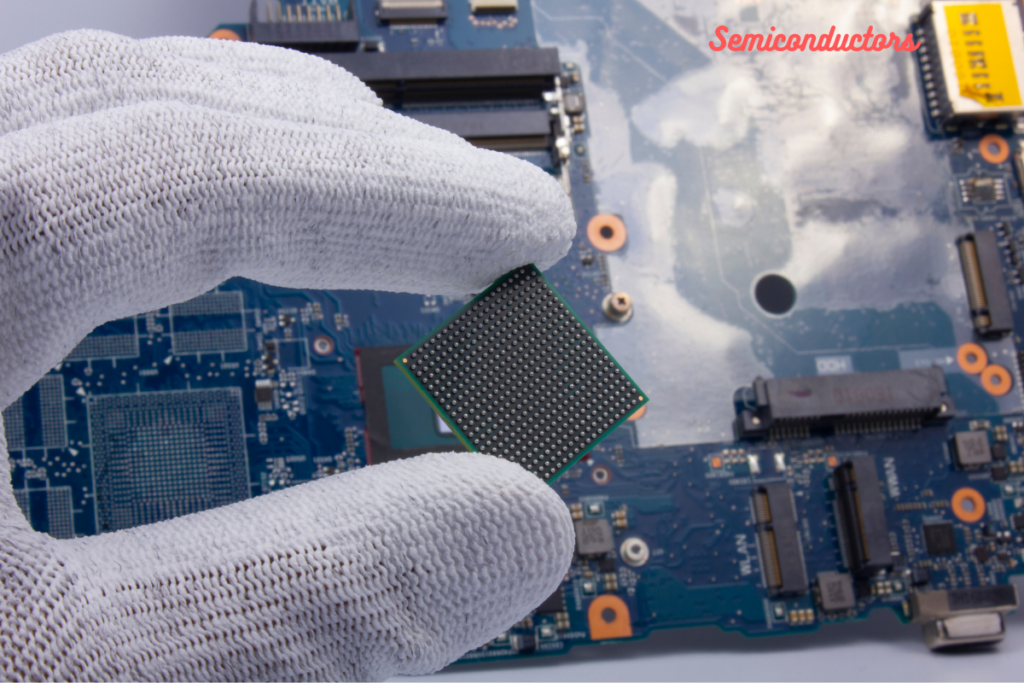
The special properties of “semiconductors” make them naturally fit for integration with conventional computer systems. Many classical devices are already based using semiconductor technology, so it becomes more realistic to include quantum components into current systems. This interoperability makes it possible to create systems that combine quantum and classical methods, therefore enabling activities benefiting from the fast calculations of quantum computers together with the dependability and built infrastructure of conventional systems. Thus, the application of “semiconductors” promotes creativity and cooperation among many domains of computation, so bridging the gap between these two paradigms.
Streamlining Hybrid Systems:
Using “semiconductors,” scientists can design hybrid systems combining the advantages of quantum and conventional computing. Where the complexity of quantum computations can complement the speed of conventional computations, these hybrid systems can maximize activities including data processing, machine learning, and cryptography. Moreover, “these material” help to build interconnects that enable efficient communication between quantum computers and classical systems, therefore simplifying data flow and improving general performance. The seamless integration of quantum and conventional technologies as “Semiconductors” develop will result in more potent computer solutions addressing a broad spectrum of issues in the present digital environment.
Scalability Challenges:
In the field of quantum computing, scalability is a major challenge since the needs for bigger and more potent quantum computers rise. In order to solve these scaling issues and guarantee that quantum computers may increase their capacity while still providing the required performance levels, researchers are investigating several approaches including “semiconductors”.
Overcoming Scalability Obstacles:
Growing complexity of quantum systems reveals various scaling issues that need to be resolved. As more qubits are added to a quantum computer, one of these difficulties is keeping coherence and reducing mistakes. Researchers are looking at “semiconductors” for their part in the construction of scalable quantum structures able to support rising qubit count without sacrificing performance. Researchers want to effectively handle numerous qubits by using cutting-edge production methods and investigating new semiconductor materials, therefore enabling quantum computers to extend their capabilities while preserving operational integrity.
Ensuring Performance and Reliability:
The success of “Semiconductors” depends mostly on their performance and dependability even as the scalability of quantum computers develops. As system sizes rise, the inherent features of “these material” can cause problems including more noise and crosstalk. Using creative methods in materials science and engineering to counteract these disadvantages is helping to produce more strong semiconductor-based qubit designs. Along with increasing qubit count, this continuous effort guarantees that performance criteria including gate fidelity and coherence time remain ideal. Making practical, large-scale quantum computing a reality will depend on these special material ongoing evolution, which will push the envelope of what these systems can accomplish.”
Development of Quantum Devices:
The fast development of quantum technologies is mostly dependent on the developments in “Semiconductors.” These materials form the basis for developing quantum devices, including quantum sensors and quantum networks, which offer transforming potential across many scientific and technical spheres.
Foundational Role in Quantum Devices:
The development of quantum devices able to sense and transmit information at hitherto unheard-of degrees of accuracy depends much on developments in “semiconductors”. For extremely sensitive detection of minute changes in physical events including magnetic fields or temperature, quantum sensors, for example, use the special characteristics of “Semiconductors”. Applications in environmental monitoring, navigation, and healthcare as well as others depend on this capacity. Using “Semiconductors,” scientists can create sensors that take use of the quantum character of particles, therefore producing tools that surpass conventional sensors in both dependability and resolution.
Facilitating Quantum Networks:
Apart from sensors, the creation and growth of quantum networks—which seek to enable safe communication and the dissemination of quantum information over vast distances—depend on the development of “semiconductors”. These networks are less prone to eavesdropping than conventional communication systems since they depend on quantum bits for safe information transfer. By enabling the integration of qubits inside fiber optics and other communication systems, innovations in “Semiconductors” help to build scalable quantum networks. As “Semiconductors” develop, they will greatly improve the capacity of quantum devices, therefore ushering in a new era of connectivity and information processing that promises to affect many areas, including national security.
Future Potential:
Constant research and invention in “Semiconductors” are opening the path for revolutionary quantum computing developments. These advances could transform several sectors and address difficult problems thought to be insurmountable in past years.
Unlocking New Possibilities:
As researchers investigate novel materials, fabrication techniques, and designs, they are finding creative ways to improve qubit performance and extend the utility of quantum systems, so intimately entwining the future of quantum computing with the developments in “semiconductors.” These developments might enable the development of more strong quantum computers capability of running intricate computations at hitherto unheard-of rates. Scientists can push the boundaries of quantum technology by using the special qualities of “semiconductors,” therefore opening doors to applications in sectors such drug discovery, financial modeling, and artificial intelligence requiring great processing capability.
Revolutionizing Industries:
Beyond the domain of quantum computing, developments in ” semiconductors” imply a significant influence on many different sectors. For example, developments in quantum algorithms made possible by better semiconductor technology could revolutionize areas including materials science, cybersecurity, and logistics, therefore enabling the creation of more effective systems and procedures. Moreover, as “Semiconductors” develop they might offer answers to some of the most urgent issues facing the planet: better communications, energy use optimization, and climate modeling enhancement. Therefore, the continuous research of “Semiconductors” not only reflects a significant area of scientific interest but also a stimulus for invention that can change our technological scene for next years.
Conclusion:
Unquestionably at the core of the quantum computing revolution, “semiconductors” are the fundamental materials driving qubit and quantum algorithm development. Their special qualities permit hitherto unheard-of control over electronic states, hence enabling the building of scalable and robust quantum systems. As scientists keep developing in the subject of “Semiconductors,” the possibility of expanding quantum technologies becomes more real with applications that could change sectors and address challenging difficulties.
The continuous developments in “Semiconductors,” which are essential for overcoming obstacles related to scalability, error correction, and device integration, will determine the direction of quantum computing most importantly. By use of hybrid systems combining classical and quantum computing, “semiconductors” not only improve the performance of quantum devices but also offer a wider spectrum of applications. As our research of “Semiconductors” advances, we stand on the edge of a new age in computing one that could significantly affect our society and result in ground-breaking ideas in many different spheres.
People Also Ask:
What are the key properties of semiconductors that make them suitable for quantum computing?
Semiconductors are ideally suited for use in quantum computing applications due to their exceptional features, which include modulable conductivity, low energy loss, and the capacity to generate qubits.
How do semiconductor materials compare to other materials used in quantum computing technologies?
Semiconductor materials provide several benefits, including flexibility, scalability, and compatibility with existing technology. On the other hand, superconductors enable faster qubit operations, but they require extremely low temperatures.
What advancements in semiconductor technology are necessary for the scalability of quantum computers?
In order to achieve scalability in quantum computers, technological advancements are required. These advancements include improved qubit coherence times, improved error correction methods, enhanced fabrication procedures, and the use of hybrid materials.